Cerro Blanco (volcano)
Cerro Blanco | |
---|---|
![]() Satellite image of Cerro Blanco volcano. The black area on the upper edge is Carachipampa volcano. Cerro Blanco caldera is located slightly left and below the centre of the image and is the gray-yellow area. | |
Highest point | |
Elevation | 4,670 m (15,320 ft) [1] |
Listing | List of volcanoes in Argentina |
Coordinates | 26°45′37″S 67°44′29″W / 26.76028°S 67.74139°WCoordinates: 26°45′37″S 67°44′29″W / 26.76028°S 67.74139°W [1] |
Naming | |
Translation | White Mountain (Spanish) |
Geography | |
![]() ![]() Cerro Blanco Location in Argentina | |
Location | Catamarca Province, Argentina |
Parent range | Andes |
Geology | |
Age of rock | Holocene |
Mountain type | Caldera |
Volcanic belt | Central Volcanic Zone |
Last eruption | 2,300 ± 160 BCE[1] |
Cerro Blanco is a caldera in the Andes of the Catamarca Province in Argentina. Part of the Central Volcanic Zone of the Andes, it is a volcano collapse structure located at an altitude of 4,670 metres (15,320 ft) in a depression. The caldera is associated with a less well defined caldera to the south and several lava domes.
The caldera has been active for the last eight million years and eruptions have created several ignimbrites. One of the most recent eruptions occurred 73,000 years ago and formed the Campo de la Piedra Pómez ignimbrite layer. About 5,000 years ago, the largest volcanic eruption of the Central Andes with a volcanic explosivity index of 7 occurred at Cerro Blanco, forming the most recent caldera as well as thick ignimbrite layers. About 110 cubic kilometres (26 cu mi) of rhyolite were erupted then. The volcano is dormant since then with some deformation and geothermal activity. A major future eruption would put local communities to the south at risk.
The volcano is also known for giant ripple marks that have formed on its ignimbrite fields. Persistent wind action on the ground has shifted gravel and sand, forming wave-like structures. These ripple marks have heights up to 1 metre (3 ft 3 in) and are separated by distances up to 30 metres (98 ft). Unlike dunes, they do not migrate with the wind and are stationary. These ripple marks are among the most extreme on Earth and have been compared to Martian ripple marks by geologists.
Geography and geology
Regional setting
The Central Volcanic Zone of the Andes is an area between 14–28° southern latitude where volcanic activity dates to the Eocene.[2] Ignimbritic activity has occurred over the whole area, particularly north of 25° southern latitude where the Altiplano–Puna volcanic complex is situated. Ignimbrites are primarily rhyolite and rhyodacite and subordinate shoshonite. The more southern ignimbrites are felsic and have a low crystal content. With the exception of the Luingo caldera and Cerro Galán, most volcanism south of 25° southern latitude is of low volume. After a phase of pyroclastic activity in the Antofalla area during the Eocene, a number of volcanoes formed from calc-alkaline magmas during the Miocene. Changes in subduction geometry including the removal of continental crust from the boundary 8-3 mya have caused a thickening of the crust as well as an eastward shift of the volcanic front.[2][3] Huaynaputina volcano in Peru is part of the Central Volcanic Zone and had a major eruption in 1600.[4]
Most ignimbrites of the southern sector have volumes of 50 cubic kilometres (12 cu mi) or less and are associated with stratovolcanoes and small calderas. They are derived from fractional crystallization of andesitic magmas with variable crustal input.[2]
Local setting
Cerro Blanco is part of the Central Volcanic Zone and is located at the southern limit of the Puna.[5] It forms a volcanic unit together with the Aguada Alumbrera ignimbrite, at the eastern edge of the Cordillera de San Buenaventura. Rocks beneath this unit include dacitic–trachyandesitic volcanites 9–7 mya old and an igneous-metamorphic granitic basement containing orthogneiss, quartz of Neoproterozoic-early Paleozoic age, containing paleozoic mafic to ultramafic volcanic inclusions. The Cordillera de San Buenaventura is formed from lava domes and volcanic complexes of intermediary composition of Upper Miocene–Quaternary age.[2][4] During the Miocene-Pleistocene, the isotope and chemical composition of areal magmas has moved away from arc like magmas to distinctly water-poor and unoxidized petrologies. This finding is consistent with a hot mantle forming after delamination of the crust and migration of the arc.[5]
Nevado Tres Cruces lies to the west and Nevado Ojos del Salado lies to the southwest of the Cerro Blanco centre. East-northeast running fault systems are controlled by local tectonics and may be responsible for the formation of the Cordillera de San Buenaventura. The 4-3.7 mya Laguna Amarga west of Cerro Blanco erupted rhyolites, unlike Cerro Blanco's ignimbrites.[5] The better known Cerro Galán caldera lies 80 kilometres (50 mi) southwest of Cerro Blanco.[1] It forms a northwest-southeast volcanic line with Cerro Blanco and Incapillo. This line was active less than 2.5 mya ago.[3] Another trend is defined by the Culampaja line running from Sierra Nevada over Cerro Cóndor and Cerro Peinado to Blanco.[6] Other volcanic centres in the region are the Miocene-Pliocene ignimbrites Las Juntas and Las Papas. Minor bimodal–mafic volcanic centres during the Pleistocene–Holocene include volcanoes in the Pasto Ventura, Salar de Carachi Pampa and Volcán Negro Peinado areals. Felsic centres include Chascón and Cueros de Purulla.[4] Cerro Purulla lies northnorthwest of Cerro Blanco and the La Hoyada volcanic complex lies directly west.[7] 4,380 metres (14,370 ft) Cueros de Purulla and 4,290 metres (14,070 ft) Chascón are major sources of obsidian in the Puna of Catamarca region.[8]
The Cerro Blanco complex is formed from pyroclastic flows extending from nested calderas. The Robledo caldera is heavily eroded and has assumed a semicircular shape. The rim of the Cerro Blanco caldera proper is well-preserved, with ash and block flows filling the interior.[2] Another theory holds that the northern Cerro Blanco and the southern Robledo calderas are part of one caldera with a minimum subsidence of 700 metres (2,300 ft) and a dimension of 13 by 10 kilometres (8.1 mi × 6.2 mi).[4] The Cerro Blanco caldera is 6 kilometres (3.7 mi) wide. On its southern rim, surrounded by ashfall deposits, lies the Holocene Cerro Blanco del Robledo lava dome (26°46′51″S 67°45′27″W / 26.78083°S 67.75750°W).[1] Some sources call the whole volcano Robledo and use Cerro Blanco to refer to the lava dome.[9] The whole volcanic complex is formed from lava domes, ignimbrites, ash and block flows and unconsolidated deposits. Cerro Blanco is the largest lava dome and has a debris collapse deposit on its northern side. A hydrothermally altered area in the middle of the caldera has been linked to phreatomagmatic activity. The El Escondido crater northeast of the caldera is 1.2 kilometres (0.75 mi) wide and 40 metres (130 ft) deep. It shows a dark colour and semiannular shape in satellite images and has been interpreted as a phreatic crater.[2] The complex is located at the southwest end of a volcano-tectonic depression formed by local fault systems. These fault systems channel magma during eruptions; usually no eruption column is formed. The formation of the depression may be influenced by the emptying of magma chambers associated with the volcano.[4] The complex is located on 60 kilometres (37 mi) thick crust.[10] A crustal low seismic velocity anomaly is located beneath Cerro Blanco, called the Southern Puna Magma Body.[11]
Megaripple fields
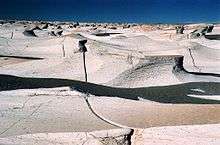
Mega ripple marks formed by gravel are also found in the Cerro Blanco area and are among the most extreme on Earth. These are formed from wind-driven processes acting on local ignimbrites, including saltation of fine grains and sand during wind. Ripples have amplitudes varying 0.1–2 metres (3.9 in–6 ft 6.7 in) and wavelengths of 10–30 metres (33–98 ft). Coarse gravel is found at their top and more fine silt in their lower parts. Pumice blocks are found in the lee surfaces of the ripples, with the interripple areas relatively flat. Unlike dunes these structures do not migrate once stably formed. The material these structures is formed from includes lithic clasts with densities 2,600–3,000 kilograms per cubic metre (4,400–5,100 lb/cu yd) and pumices with densities 800–1,300 kilograms per cubic metre (1,300–2,200 lb/cu yd). The formation of the ripples is associated with bedrock ridges. These ripple marks have been compared to Martian ripple fields.[7][12]
The largest field is named Campo Piedra Pómez (centered 26°36′17.30″S 67°34′50.46″W / 26.6048056°S 67.5806833°W) and covers a surface area of 600 square kilometres (230 sq mi), descending from 3,800 metres (12,500 ft) at La Hoyada in the south to 3,000 metres (9,800 ft) Carachipampa volcano in the north. The megaripples in this field are dark, formed from older ignimbrites and lavas of the 70,000-year-old Piedra Pómez ignimbrite. Northwest of Cerro Blanco caldera and towards Laguna Purulla lies another field (centered 26°39′49.97″S 67°48′55.83″W / 26.6638806°S 67.8155083°W) with a surface area of 127 square kilometres (49 sq mi), It has a dark colour from andesitic lava and falls down from 4,100 metres (13,500 ft) altitude at La Hoyada to 3,700 metres (12,100 ft) at Laguna Purulla. Reddish-brown Rosada ignimbrites and white basement material are also components of this field. Other fields are the 12 square kilometres (4.6 sq mi) Campo Purulla (centered 26°37′12.27″S 67°46′00.66″W / 26.6200750°S 67.7668500°W) field downslope of Cerro Purulla with a distinct albedo from the 13,000 years old Purulla ignimbrite, and the 54 square kilometres (21 sq mi) Salar de Incahuasi (centered 26°31′53.61″S 67°41′36.93″W / 26.5315583°S 67.6935917°W) field from the Piedra Pómez ignimbrite of a brown colour.[7][12]
Climate
The Puna climate in the area is a high desert climate with high insolation and long-term aridity.[13] The desert environment of the Puna results in low levels of erosion.[4] Plant growth is minor. Wind speeds of 33 kilometres per hour (21 mph) are regularly found and may play a role in the formation of megaripples.[7] While there are no meteorological data for the Piedra Pómez area, a similar climate is found in Salar del Rincón where in 2014 temperatures −19.6–22.9 °C (−3.3–73.2 °F) were recorded. Recorded precipitation amounted to 7.1 millimetres (0.28 in) with evaporation rates of 764.5 millimetres (30.10 in).[13]
A weather station (26°28′58.6″S 67°41′1.3″W / 26.482944°S 67.683694°W) was run from March to November 2013 in the Salar de Incahuasi, at 3,300 metres (10,800 ft) altitude. Winds recorded there are mostly northwestern and southwestern and have gusts of >59 kilometres per hour (37 mph) every month. Stronger average winds are found during spring.[12]
Eruptive history
Human research in the San Buenaventura area goes back to Stelzner 1885, mineral-focused research in 1895 and Penck 1920. The La Hoyada Formation was identified 1963 and reinterpreted as the La Hoyada volcanic complex in 2000. General geological research in the region occurred 1988, 2000 and 2006, and research focused on Cerro Blanco was done by Arnosio et al. 2005 and 2008.[2]
Activity of Cerro Blanco goes back 8 mya, when the Maricunga Belt in the west was active.[5] The volcano is responsible for a large Holocene eruption with a Volcano explosivity index 7, the largest in the Andean Central Volcanic Zone for 5000 years. It is comparable to Santorini and Tambora's large eruptions.[14]
Pre-Holocene
The Aguada Alumbrera Ignimbrite is a white-rosa ignimbrite composed from two units, 7.14 mya Unidad Blanquecina and 13.57 mya Unidad Rojiza. The Blanquecina unit is of white to white-rosa colour and contains amphibole, biotite and quartz crystals. It contains lithic fragments of dacitic composition. The groundmass of the ignimbrite is grey in colour and contains glass and phenocrysts. Next to Laguna Aguada Alumbrera it is 100 metres (330 ft) thick. The ignimbrite is unconformably overlaid by ignimbrites from La Hoyada volcano and overlies the basement. There are fluvial deposits contained within the Blanquecina ignimbrite, suggesting that sedimentation occurred between the eruptions. The other subunit Rojiza is of white colour and is rich in biotite and quartz. It is mostly buried by other deposits; south of Aguada Alumbrera the principal deposit is 40 metres (130 ft) thick.[2] This ignimbrite crops out south of Cerro Blanco.[10]
The Rosada Ignimbrite is located next to Cueros de Purulla and has a rosa colour, due to fiamme formed from welded pumice. The pumice contains biotite, hornblende and quartz. It also contains lithic fragments from the basement and from vulcanites. Two dates of 6.3 and 7.3 mya have been obtained; the younger age is likely the result from argon loss and less accurate.[2] It and the Aguada Alumbrera ignimbrites are coeval but different compositions indicate that they derive from separate eruptions.[10] The Rosada ignimbrite's main minerals are biotite, quartz, plagioclase and sanidine. It has a volume of 50 cubic kilometres (12 cu mi).[15]
A lava dome (26°40.26′S 67°45.90′W / 26.67100°S 67.76500°W) formed 7.3 ± 0.5 mya and red ignimbrite 8.1 ± 0.5 mya. 2.3–1.3 mya an east-northeast chain of andesitic lava domes was formed, with the youngest dome (26°40.63′S 67°45.1′W / 26.67717°S 67.7517°W) being 1.3 ± 0.4 mya old and located next to Cerro Blanco. 0.8 mya mafic andesite was erupted south and west of Carachipampa (26°28.251′S 67°24.94′W / 26.470850°S 67.41567°W) and Laguna de Purulla (26°39.3′S 67°52′W / 26.6550°S 67.867°W). The Cueros de Purulla obsidian lava dome (26°34.06′S 67°44.97′W / 26.56767°S 67.74950°W) formed 0.4 ± 0.1 mya.[5]
The Barranca Blanca and Carachi Ignimbrites are covered by lavas from Volcán Carachipampa and are thus older than 0.75 mya. They contain pumices of various colours and lithic fragments, including mylonite and schist. The Barranca Blanca ignimbrite is white in colour and contains rhyodacitic white pumice with subordinate amounts of grey trachyandesitic pumice. The Carachi Ignimbrite overlies the Barranca Blanca ignimbrite. It is weakly welded and contains white pumice and crystals with fiamme. Both of these are pyroclastic density currents that were still hot at the time of deposition.[4]
The Campo de la Piedra Pómez ignimbrites (26°39′S 67°43′W / 26.65°S 67.71°W) have a surface area of 261.83 square kilometres (101.09 sq mi).[3] They have a volume of 17 cubic kilometres (4.1 cu mi) and are separated in two layers which form one cooling unit despite their different colour and mineral composition. They both contain pumice and lithic fragments and are poor in crystals. Both have a pyroclastic texture with pumices bearing a porphyritic texture. Dating techniques yield different ages; the most reliable one (argon–argon dating on sanidine) indicates that these ignimbrites were erupted 73,000 ± 23,200 years ago.[4] Other proposed dates go as far back as 0.555 ± 0.11 mya.[2] They do not cross topographic surfaces and were generated by tranquil release from the ground rather than through the collapse of an eruption column. The surface has been exposed to wind erosion, forming yardangs and ripple marks. The source of this eruption is unknown; the Robledo caldera may be the origin of this eruption but this is controversial.[4][7] The ignimbrites of Piedra Pómez are covered with a desert varnish similar coating, some bearing signs of endolithic lifeforms.[13]
Holocene
2,300 ± 160 BCE a large Plinian eruption occurred at Cerro Blanco. Rhyolites with a volume of 110 cubic kilometres (26 cu mi) generated ashfalls and ignimbrites, forming the largest known eruption in the Central Andes during the Holocene.[1] These Purulla/El Médano ignimbrites (26°51′S 67°43′W / 26.85°S 67.72°W) cover an area of 51.61 square kilometres (19.93 sq mi)[3] and are dated to have occurred less than 12,200 years ago on the basis of radiocarbon analysis of organic material contained between various ignimbrite layers. One layer in La Hoyada is dated between 8,830 and 5,480 BP.[2] Other data attribute the Purulla ignimbrites to 22,000 years ago.[16] The date of the major Holocene eruption is constrained to between 5,500 and 4,000 years ago. Based on stratigraphic relationships, the Cerro Blanco caldera formed during this eruption. The location of the vent did not change during the course of the eruption, indicating that the annular fractures around the caldera were possibly uninvolved in the process.[4] The low population density in the Fiambalá valley south of the volcano during the 10,000–3,000 B.P interval is ascribed to this eruption.[17]
The ignimbrites from the caldera-forming eruption have a volume of 17 cubic kilometres (4.1 cu mi) and cover a surface area of 527 square kilometres (203 sq mi) south, north and east of the caldera.[4] They are partly welded and contain fiamme and lithic fragments. The material is vitreous and may have been fluid at the time of deposition. Breccia derived from column collapse are also found. The deposits are microcristalline with much glass. A few kilometres from the calderas they abruptly turn into medium-sized pumice deposits. Some ignimbrites from this stage are derived from the lava domes placed within small depressions, the largest of which is 1.3 kilometres (0.81 mi) wide.[2] Aeolian erosion has reworked the ashes generated by the eruption, generating dunes.[4]
The Purulla/El Médano ignimbrites have a precaldera lower layer that various methods have dated 20,000 to 5,000 years ago. This stage was effusive and accompanied by the emplacement of lava domes. A postcaldera stage is represented by lava domes on the southeast edge of the caldera, as well as block and ash flows inside the caldera. These are rhyolitic in composition. Their age is unknown, but postdates the caldera formation.[4]
Recent unrest and threats
There are no reports of historical activity.[2] A small geothermal field is associated with the volcanic complex, consisting of fumaroles, mud volcanoes and thermal anomalies.[16]
The caldera floor has been subsiding in the 1990s according to satellite images.[1] The diametre of the deformation region is less than 30 kilometres (19 mi) and the deformation occurs at depths of less than 4.8 kilometres (3.0 mi).[18] The deflation speed has been decreasing from more than 2.5 centimetres per year (0.98 in/year) between 1992 and 1997 over 1.8 centimetres per year (0.71 in/year) between 1996 and 2000 and 1.2 centimetres per year (0.47 in/year) between 2003 and 2007 to 0.87 centimetres per year (0.34 in/year) between 2005 and 2010. This deformation is centered between the Robledo and Cerro Blanco calderas and is accompanied by an uplift to the northwest. The deflation has been interpreted to depend on a magma chamber with a diametre of 17 kilometres (11 mi) but is unlikely to be caused by magma withdrawal[16] or tectonic expansion. It may be linked to hydrothermal effects however.[9] A seismic swarm was noted at 15 kilometres (9.3 mi) depth in 2007 and 2009.[2]
Evidence indicates that such calderas can fill in within a timespan of hundreds to thousands of years. Two possible scenarios of an eruption are the formation of Plinian eruption columns or more tranquil subhorizontal eruption of pyroclastic flows from vents. Given the low population density of 0.06 people per 1 square kilometre (0.39 sq mi), the impact of the eruption on local population will be small. Westerly winds may cause larger impact west of the centre, with air travel particularly affected. The community of Bolsón de Fiambalá 50 kilometres (31 mi) south of the caldera is beneath the level of Cerro Blanco and connected to it by valleys. In a future eruption, pyroclastic currents and less dense clouds associated with them from Cerro Blanco might reach the community.[4]
See also
References
- 1 2 3 4 5 6 7 "Cerro Blanco". Global Volcanism Program. Smithsonian Institution. Retrieved 14 September 2015.
- 1 2 3 4 5 6 7 8 9 10 11 12 13 14 López, María; Hongn, Fernando; Brod, José; Seggiaro, Raúl; Marrett, Randall; Sudo, Masafuni (November 2010). "Magmatismo ácido del mioceno superior-cuaternario en el área de Cerro Blanco-La Hoyada, Puna Austral". Revista de la Asociación Geológica Argentina (in Spanish). Buenos Aires: SciELO. 67 (3). ISSN 0004-4822. Retrieved 14 September 2015.
- 1 2 3 4 Guzmán, Silvina; Grosse, Pablo; Montero-López, Carolina; Hongn, Fernando; Pilger, Rex; Petrinovic, Ivan; Seggiaro, Raúl; Aramayo, Alejandro (December 2014). "Spatial–temporal distribution of explosive volcanism in the 25–28°S segment of the Andean Central Volcanic Zone". Tectonophysics. 636: 170–189. Bibcode:2014Tectp.636..170G. doi:10.1016/j.tecto.2014.08.013. Retrieved 14 September 2015.
- 1 2 3 4 5 6 7 8 9 10 11 12 13 14 Walter Báez, Marcelo Arnosio, Agostina Chiodi, Agustín Ortíz-Yañes, José Germán Viramonte, Emilce Bustos, Guido Giordano, José Francisco López (2015). "Estratigrafía y evolución del Complejo Volcánico Cerro Blanco, Puna Austral, Argentina" (PDF). Revista Mexicana de Ciencias Geológicas (in Spanish). National Autonomous University of Mexico. 32 (1): 29–49. Retrieved 14 September 2015.
- 1 2 3 4 5 Suzanne Mahlburg Kay; B. Coira; Constantino Mpodozis (August 2006). "Late Neogene volcanism in the Cerro Blanco region of the Puna Austral Argentina, (~26.5°S, ~67.5°W)". Volcanismo y Magmatismo. XI Congreso Geológico Chileno. Antofagasta: ResearchGate. pp. 499–502. Retrieved September 14, 2015.
- ↑ Mulcahy, Patrick; Chen, Chen; Kay, Suzanne M.; Brown, Larry D.; Isacks, Bryan L.; Sandvol, Eric; Heit, Benjamin; Yuan, Xiaohui; Coira, Beatriz L. (August 2014). "Central Andean mantle and crustal seismicity beneath the Southern Puna plateau and the northern margin of the Chilean-Pampean flat slab". Tectonics. 33 (8): 1636–1658. Bibcode:2014Tecto..33.1636M. doi:10.1002/2013TC003393. Retrieved 15 September 2015.
- 1 2 3 4 5 de Silva, S. L.; Spagnuolo, M. G.; Bridges, N. T.; Zimbelman, J. R. (2013). "Gravel-mantled megaripples of the Argentinean Puna: A model for their origin and growth with implications for Mars". Geological Society of America Bulletin. 125 (11–12): 1912–1929. doi:10.1130/B30916.1. ISSN 0016-7606.
- ↑ Yacobaccio, Hugo D; Escola, Patricia S; Pereyra, Fernando X; Lazzari, Marisa; Glascock, Michael D (February 2004). "Quest for ancient routes: obsidian sourcing research in Northwestern Argentina". Journal of Archaeological Science. 31 (2): 193–204. doi:10.1016/j.jas.2003.08.001. Retrieved 15 September 2015.
- 1 2 Pritchard, M. E.; Simons, M. (February 2004). "An InSAR-based survey of volcanic deformation in the central Andes". Geochemistry, Geophysics, Geosystems. 5 (2): n/a–n/a. Bibcode:2004GGG.....5.2002P. doi:10.1029/2003GC000610. Retrieved 15 September 2015.
- 1 2 3 Montero Lopez, M.C.; Hongn, Fernando D.; Strecker, Manfred R.; Marrett, Randall; Seggiaro, Raúl; Sudo, Masafumi (October 2010). "Late Miocene–early Pliocene onset of N–S extension along the southern margin of the Central Andean Puna Plateau: Evidence from magmatic, geochronological and structural observations". Tectonophysics. 494 (1–2): 48–63. Bibcode:2010Tectp.494...48M. doi:10.1016/j.tecto.2010.08.010. Retrieved 14 September 2015.
- ↑ Bianchi, M.; Heit, B.; Jakovlev, A.; Yuan, X.; Kay, S.M.; Sandvol, E.; Alonso, R.N.; Coira, B.; Brown, L.; Kind, R.; Comte, D. (February 2013). "Teleseismic tomography of the southern Puna plateau in Argentina and adjacent regions". Tectonophysics. 586: 65–83. Bibcode:2013Tectp.586...65B. doi:10.1016/j.tecto.2012.11.016. Retrieved 20 September 2015.
- 1 2 3 Bridges, N.T.; Spagnuolo, M.G.; de Silva, S.L.; Zimbelman, J.R.; Neely, E.M. (June 2015). "Formation of gravel-mantled megaripples on Earth and Mars: Insights from the Argentinean Puna and wind tunnel experiments". Aeolian Research. 17: 49–60. Bibcode:2015AeoRe..17...49B. doi:10.1016/j.aeolia.2015.01.007. Retrieved 15 September 2015.
- 1 2 3 Aulinas, M.; Garcia-Valles, M.; Fernandez-Turiel, J. L.; Gimeno, D.; Saavedra, J.; Gisbert, G. (30 March 2015). "Insights into the formation of rock varnish in prevailing dusty regions". Earth Surface Processes and Landforms. 40 (4): 447–458. Bibcode:2015ESPL...40..447A. doi:10.1002/esp.3644. Retrieved 15 September 2015.
- ↑ Gertisser, R.; Self, S. (July 2015). "The great 1815 eruption of Tambora and future risks from large-scale volcanism". Geology Today. 31 (4): 132–136. doi:10.1111/gto.12099. Retrieved 14 September 2015.
- ↑ Kay, Suzanne Mahlburg; Coira, Beatriz L.; Caffe, Pablo J.; Chen, Chang-Hwa (2010). "Regional chemical diversity, crustal and mantle sources and evolution of central Andean Puna plateau ignimbrites". Journal of Volcanology and Geothermal Research. 198 (1–2): 81–111. Bibcode:2010JVGR..198...81K. doi:10.1016/j.jvolgeores.2010.08.013. ISSN 0377-0273.
- 1 2 3 Brunori, C.A.; Bignami, C.; Stramondo, S.; Bustos, E. (August 2013). "20 years of active deformation on volcano caldera: Joint analysis of InSAR and AInSAR techniques". International Journal of Applied Earth Observation and Geoinformation. 23: 279–287. Bibcode:2013IJAEO..23..279B. doi:10.1016/j.jag.2012.10.003. Retrieved 15 September 2015.
- ↑ Ratto, Norma; Montero, Carolina; Hongn, Fernando (September 2013). "Environmental instability in western Tinogasta (Catamarca) during the Mid-Holocene and its relation to the regional cultural development". Quaternary International. 307: 58–65. doi:10.1016/j.quaint.2012.09.014. Retrieved 15 September 2015.
- ↑ Caricchi, Luca; Biggs, Juliet; Annen, Catherine; Ebmeier, Susanna (February 2014). "The influence of cooling, crystallisation and re-melting on the interpretation of geodetic signals in volcanic systems". Earth and Planetary Science Letters. 388: 166–174. Bibcode:2014E&PSL.388..166C. doi:10.1016/j.epsl.2013.12.002. Retrieved 15 September 2015.
External links
- Montero López, M.C.; Hongn, F.; Seggiaro, R.; Marrett, R.; Ratto, N. (June 2013). Relación entre el volcanismo y los registros arqueológicos en el bolsón de Fiambalá (Departamento Tinogasta, Catamarca) (in Spanish). Buenos Aires: EUDEBA. pp. 131–158.
- "Robledo ASTER Imagery". Aster Volcano Archive. NASA. Archived from the original on 10 September 2015. Retrieved 15 September 2015.